서 론
출생 후 동물 소화장관의 발달은 다양한 자극에 의해 조절되며 동시에 장관면역의 발달도 일어난다. 장관면역은 동물의 출생 전부터 발달하기 시작하여 출생 후에도 연령에 따라 계속적인 발달이 진행되며, 이러한 장관면역의 발달은 소화 장관 내 다양한 환경변화에 의한 자극에 의해 조절된다고 보고되고 있다(Schmaler et al., 2018). 장관면역 발달 조절에 중요한 역할을 담당하는 것 중 하나가 장내 미생물이다. 예를 들어 부화한 닭의 소화장관은 신생기 발달과정 동안 장내 미생물군의 군집화가 이루어지며, 이러한 장내 미생물의 정착이 장관면역의 중요 조절 인자임이 보고되고 있다(Rubio, 2019). 동물의 장관에서 서식하는 장내 미생물 군집은 동물의 대사작용에 다양한 방식을 통해 영향을 미쳐 경제동물의 생산성과 밀접한 연관이 되어 있음이 보고된 바 있다(Borda-Molina et al., 2018). 또한 장내 미생물은 동물의 건강 및 면역에도 영향을 미친다(Sethi et al., 2018). 닭에서의 장내 미생물의 다양성은 생후 1주일 동안 가장 증가하는 경향을 가지는데(Rubio, 2019), 신생동물의 유익한 장내 미생물 군집 정착은 동물의 전체 수명에 큰 영향을 미치며, 건강상태나 면역 반응과도 연관되어 있음이 다른 경제동물을 통해 보고되고 있다(Malmuthuge et al., 2015; Guevarra et al., 2019). 예를 들어 가금류에서 장내 미생물의 초기 정착은 미생물 감염 및 면역력 증진과 관련되어 있다(Kers et al., 2018).
그러므로 신생 경제동물은 장관면역이 완전히 발달하기 전까지 다양한 미생물 감염에 매우 취약한 상태이다. 신생동물의 질병을 예방하기 위해 다양한 항생제가 경제동물을 대상으로 사용되어 왔으나 근래 강력한 항생제 사용 규제 정책이 실시되면서 다양한 면역 증강 항생제 대체제 개발이 필요한 상태이다. 항생제 대체제로 사용되는 다양한 첨가제는 경제동물에 긍정적인 영향을 나타내는 것으로 알려져 있다. 예를 들어 이유자돈에게 항생제 대체제로써 프로바이오틱스 중 하나인 Duan-Nai-An을 첨가하였을 때 장내세균총을 조절하고, 설사의 발생률을 감소시켰다고 보고되었다(Xu et al., 2018). 또한 닭에게 첨가제로써 구기자를 급여하였을 때 면역력 및 항산화 능력이 증가되었으며(Long et al., 2020), 특히 신생육계에게 사료 첨가제를 급여하였을 때 대사장애 확률을 낮춰주고, 병원체에 대한 저항력을 증가시켜 면역력이 증강되는 효과가 있음이 보고되었다(Shehata et al., 2021).
경제동물 대상의 첨가제로 활용 가능한 다양한 소재 중 하나로 미세조류는 그 활용가치가 높을 것으로 보인다. 미세조류는 주로 담수나 해양에서 광합성을 통해 유기물을 생산해내는 조류로써 현재 10만종 이상이 알려져 있다. 탄수화물, 단백질, 지질을 풍부하게 가지고 있으며, 대기 중 산소의 약 50% 생산을 수행할 수 있는 필수적인 미생물이다. 미세조류는 오메가-3, 베타글루칸, 아스타잔틴 등의 고부가가치의 기능성 물질로써 가치를 가진다(Kwak et al., 2021). 그렇기 때문에 미세조류 및 그 추출물은 인간을 위한 건강보조식품의 원료로 사용될 뿐 아니라 경제동물을 위한 기능성 첨가제 재료로써 활용 가능성을 보여주고 있다.
닭의 사료에 미세조류를 첨가해주었을 때 건강, 면역, 생산성 등이 개선되었음이 보고된 바가 있다(El-Bahr et al., 2020). 특히 가금류의 사료에 chlorella를 첨가해주었을 때 장내 미생물의 다양성이 증가되었으며(Abdelnour et al., 2019), 질병에 대한 저항성에도 영향을 미칠 수 있다고 보고되었다(Kwak et al., 2011; Chen et al., 2019). 또한, 미세조류 첨가 시 염증성 사이토카인으로 알려진 IL-6의 발현을 억제시켜 항염증 효능을 보인다는 것도 보고된 바 있다(Chang et al., 2021). 산란계에서 미세조류의 첨가는 산란계의 달걀껍질 두께를 증가시키고 난황의 콜레스테롤 함량을 감소시킨다는 것이 보고되었다(Ginzberg et al., 2000). 본 연구에 사용된 Tetradesmus obliquus(TO)는 다른 미세조류와 마찬가지로 항산화, 항암, 항고혈압 및 항균활성과 같은 다양한 생물학적 기능을 가지고 있다고 보고되었다(Giordano et al., 2018). 미세조류 급여가 동물의 생리대사에 미치는 다양한 효과가 보고된 바 있으나 아직까지 사료 내 미세조류(TO) 첨가가 가금의 장관면역 또는 장내 미생물에 미치는 영향에 관한 연구는 부족한 실정이다. 따라서 본 연구에서는 초기 육계 사료 내 미세조류(TO) 첨가가 장관면역 성상과 장내 미생물에 미치는 영향을 규명하고자 하였다. 본 연구를 통해 초기 육계 사료 내 미세조류 첨가가 장관 내 T cell 분포에 영향을 미치며, 장내 미생물 균총의 다양성을 증가시킬 뿐 아니라 일부 장내 미생물을 증가시키는 것을 확인하였다. 본 연구는 미세조류가 초기 육계의 장관면역과 장내 미생물 균총 조절에 효능을 가짐을 제시하고 있다.
재료 및 방법
본 연구의 동물실험은 경북대학교 동물실험윤리위원회(IACUC) 규정에 따라 진행되었다(승인번호:2021-0213).
본 연구에 사용된 미세조류 분리, 배양 및 건조는 이전 연구에 사용된 방법을 이용하여 진행되었다(An et al., 2020). 담수 시료로부터 미세조류를 분리 및 확보하기 위하여 광학현미경을 이용하여 단일 미세조류 종을 분리하였으며 18S rDNA sequencing 분석을 실시하여 미세조류(TO)임을 확인하였다.
미세조류를 배양하기 위하여 BG-11 배지(NaNO3 1.5 g/L, K2HPO4 0.04 g/L, MgSO4·7H2O 0.075 g/L, CaCl2·2H2O 0.036 g/L, Citric acid 0.06 g/L, Ferric ammonium citrate 0.06 g/L, EDTA(disodium magnesium salt) 0.01 g/L, Na2CO3 0.02 g/L, Trace-metal mix A5(H3Bo3 2.86 g, MnCl2·4H2O 1.81 g, ZnSO4·7H2O 0.22 g, Na2MoO4·2H2O 0.39 g, CuSO2·5H2O 0.079 g, CO (NO3)2·6H2O 0.0494 g/L)를 121°C, 1.5기압에서 15분간 멸균한 뒤 사용하였다.
Tetradesmus 균체의 대량 배양은 이전 실험과 동일하게 진행하였다. 간략히 기술하면, 100 L 평판형광생물반응기에 통기(aeration)가 가능하도록 설치하여, 5% 이산화탄소 가스가 함유된 공기를 0.1 vvm으로 주입하였다. 배양온도는 22±1°C로 설정하고, 광도의 경우 500 kW 백색(6,000K) LED 광패널 2기를 이용하여 연속적으로 공급하였다. 약 7~10일간 배양한 후 원심분리기를 이용하여 미세조류 균체를 회수한 뒤, 동결건조(FD8512, Ilshinbiobase Co. Ltd., Dongducheon, Korea)를 진행하였다. 동결건조 된 미세조류는 실험에 사용시까지 자동습도조절장치(KA33-73, Sanplatec Corp., Osaka, Japan)에 보관하였다.
초생추인 육계(Ross 308) 수병아리 120수를 사용하여 동물실험을 진행하였다. 초생추는 부화 후 10일간 사양시험을 실시하였으며, 계사 내 온도는 33°C 습도 40~50%, 점등 24시간 연속 점등 조건에서 사육하였다. 시험에 사용된 사료는 기초사료에 미세조류(TO) 0.5%(옥수수전분 대체)가 첨가된 총 2종의 배합사료로 구성되었고(Table 1), 사료 내 영양소 및 아미노산 함량 계산치에 대한 정보는 Table 2와 같으며, 비타민 및 광물질 함량은 NCR(2014)에 제시되어 있는 요구량 추정치 이상이 되도록 배합하였다. 실험설계는 난괴법을 사용하여 실험 개시일에 실험에 공시된 전체 병아리를 개별 인식표 부착 후 개별 체중을 기준으로 처리당 2개의 그룹으로 나누었다. 이전에 보고된 연구방법을 참고하여 2처리 6반복 당, 10수를 배치하였다(Kim and Lindemanna, 2007; An et al., 2020).
육계 소장 고유층 세포의 분리를 위하여 CO2 호흡마취로 안락사 후, 소장 중 하나인 공장 조직을 수집하였다. 소장 고유층 세포의 분리는 이전에 보고된 방법에 따라 시행하였다(Davies and Parrott, 1981). 육계의 공장조직을 분리하여 10% fetal bovine serum(FBS; Gibco BRL, Burlington, ON, Canada), 1% penicillin-streptomycin(P/S; HyClone Laboratories, Logan, UT)이 첨가된 RPMI 1640(Gendepot, Barker, TX, USA) 배지(cRPMI)가 담긴 용기로 옮기고, cell debris를 제거하기 위해 장간막을 제거하였다. 장의 장축을 따라 가위로 자르고 소장을 핀셋으로 잡아 차가운 인산완충생리식염수(Phosphate buffered saline, PBS; Gibco, Life Technologies, São Paulo, Brazill)에 3회 세척하여 소장의 내용물을 제거한 후 1.5-2.0 cm의 길이로 잘랐다. 이 조직을 PBS에 2분간 세척하고, 세포분리용액(1 mM DL-Dithiothreitol(DTT; Sigma-Aldrich, Irvine, UK), 30 mM ethylenediamine-tetraacetic acid (EDTA; Thermo Fisher Scientific/Ambion, Waltham, Massachusetts), 10mM 4-[2-hydrxyethyl]-1-piperazineethanesulfonic acid(HEPES; Thermo Fisher Scientific, Waltham, MA, USA)이 첨가된 PBS에 넣고, 진탕배양기(200 rpm, 37°C, 10분)를 작동하였다. 세포분리용액이 담긴 PBS에서 1분간 세척한 후, DTT를 제외한 세포분리용액(30 mM EDTA, 10 mM HEPES)이 첨가된 PBS에 조직을 넣고 진탕배양기(200 rpm, 37°C, 10분)를 사용하여 장상피조직이 분리되도록 하였다. 세포분리용액이 담긴 PBS에서 1분간 세척한 뒤, 엉켜있는 조직들을 풀어주었다. cRPMI 배지에 조직을 넣고 2분간 약하게 세척한 후, 0.5 mg/mL collagenase type VIII(Sigma-Aldrich, Chem, USA)가 첨가된 cRPMI 배지를 사용하여 60분간 효소 처리를 하고, 1분간 세척하였다. 이후 원심분리기(800g, 20~22°C, 5분)를 사용하여 상층액을 분리하고 흡입기로 제거하였다. 그리고 세포부유액 40%(밀도 1.058 g/mL)/70%(밀도 1.093 g/mL) Percoll(GE Healthcare/Amersham, Bucking-hampshire, UK)를 사용하여 세포의 밀도차에 따라 고유층의 면역세포를 분리한 후, 유세포 분석에 사용하였다.
분리한 공장 고유층 면역 세포를 FACS Canto II(Becton Dickinson, Heidelberg, Germany)를 사용하여 측정하였으며, FlowJo software v10.7.1(Tree Star Inc., OR, USA)를 사용하여 분석하였다. 각 항체들은(1:200)으로 PBS에 희석하여 염색하였고, 살아있는 세포만 분석하기 위하여 LIVE/DEAD™ Fixable aqua dead cell stain kit(Invitrogen, Thermo Fisher Scientific)를 (1:1000)으로 PBS에 희석하여 항체와 함께 염색하였다. 염색된 세포들은 차광상태로 30분간 반응시키고, 원심분리기(800g, 4°C, 5분)를 사용하여 분리된 상층액을 흡입기로 제거하였다. 세포를 고정시키기 위해 4% phosphate-buffered formallin(4% PFA; Biosesang Co., Ltd, Republic of Korea)를 200 μL 사용하였고, 차광상태로 4°C 냉장고에 측정전까지 보관하였다. 본 연구에서 사용한 항체는 소장 내 대식세포와 B 세포를 확인하기 위하여 FITC-conjugated mouse anti chicken MHC II(8350-02, SouthernBiotech Birmingham, AL, USA), Alexa Fluor 647-conjugated mouse anti-chicken Bu-1(8395-31, SouthernBiotech, LA, USA), PE-conjugated mouse anti-chicken Mono/Macro(8420-09, SouthernBiotech, LA, USA) 항체를 이용하였다. 소장 내 T 세포를 확인하기 위해 Pacific Blue-conjugated mouse anti-chicken CD3(8200-26, SouthernBiotech, LA, USA), FITC-conjugated mouse anti-chicken CD4(8210-02, SouthernBiotech, LA, USA), SPRD-conjugated mouse anti-chicken CD8α(8220-13, SouthernBiotech, LA, USA), PE-conjugated mouse anti-chicken TCRγδ(8230-09, SouthernBiotech, LA, USA)을 이용하였다.
사료 내 미세조류 첨가 효과에 따른 장 항상성을 분석하기 위하여 장관 내 존재하는 IgA를 조사하였다. 종료일(11일)에 육계의 분변을 수집하여 분석 전까지 초저온 냉동고(−80°C)에 보관하였다. 수집된 분변은 PBS를 사용하여 0.1 g/mL로 희석하고, vortex mixer를 사용하여 분쇄하였다. 이후 원심분리기(7,000 g, 4°C, 15분)를 사용하여 상층액만 수집하고 ELISA 측정전까지 냉동고(−20°C)에 보관하였다. ELISA 방법은 닭 IgA 항체 측정용 ELISA kit(CUSABIO Technology, Houston, USA)을 사용하였으며, 제조회사의 방법대로 실시하였다. AMR-100 Microplate reader(Bioand, Namyangju, Korea)를 사용하여 450 nm에서 흡광도를 측정하고, 육계 분변 내 IgA의 양을 상대 정량하였다.
사료 내 미세조류 첨가 효과에 따른 장내 미생물 변화를 확인하기 위해 CO2 호흡마취로 안락사 후, 맹장 내 분변을 수집하였다. 수집된 모든 시료들은 DNA 추출과 장내 미생물 군집 분석을 위해 초저온 냉동고(−80°C)에 보관하였다.
DNA는 DneasyPowerSoil Kit(Qiagen, Hilden, Germany)를 사용하여 제조사의 프로토콜을 따라 진행하였다. 추출된 DNA는 Quant-IT PicoGreen(P7589, Invitrogen)을 사용하여 정량화하였다. 장내 미생물 분석을 위한 이후의 과정은 일루미나(Illumina Inc.)에서 제공하는 16S Metagenomic sequencing library 프로토콜에 따라 진행하였다. Amplicon PCR 과정에서 16S rRNA gene의 V3-V4 region을 target으로 진행하였으며, 사용된 primer의 염기서열로 V3은 5’-TCG TCG GCA GCG TCA GAT GTG TAT AAG AGA CAG CCT ACG GGN GGC WGC AG-3’(forward primer), V4는 5’-GTC TCG TGG GCT CGG AGA TGT GTA TAA GAG ACA GGA CTA CHV GGG TAT CTA ATC C-3’ (reverse primer)로 마크로젠(Macrogen, Inc., Seoul, Korea)에서 제작하여 사용하였다. 제작된 library는 마크로젠에 위탁하여 Miseq(Illumina, San Diego, USA)을 진행하였다. Amplicon sequencing 과정의 오류를 교정하기 위해 R(v4.0.3) 프로그램의 DADA2(v1. 18.0) package를 사용하였고, Paired-end Read를 대상으로 Forward 서열(Read1)과 Reverse 서열(Read2)을 각각 250 bp, 200 bp로 자르고, expected errors가 2 이상인 서열은 제외하였다. 이후, Batch 별 Error model을 수립하여 샘플별 노이즈를 제거하였다. 이렇게 sequencing error가 교정된 paired-end 서열을 하나의 서열로 조립한 뒤, DADA2의 Consensus method를 사용하여 Chimera 서열을 제거하고 ASVs를 형성하였다. 아울러, 미생물 군집 비교 분석을 위해 QIIME(v1.9) 프로그램을 이용하여 전체 샘플 중 최소 read 수를 가지는 샘플의 read 수를 기준으로 subsampling을 적용하여 정규화하였다. 각 ASVs 서열은 Reference DB(NCBI 16S Microbial DB)에 BLAST+(v2.9.0)를 수행하여, 유사성이 가장 높은 subject의 organism에 대한 taxanomy 정보를 할당하였다. 이때, DB에 매치되는 best hit의 Query coverage가 85% 미만이거나, 매치된 영역의 identity가 85% 미만이면 taxanomy 정보를 할당하지 않는다. 또한, ASVs 서열 간의 multiple alignment를 위해 mafft(v7.475) 프로그램을 사용하였으며, ASVs abundance와 taxonomy 정보로 QIIME을 이용하여, 다양한 장내 미생물 군집 비교 분석을 수행하였다. 샘플 내 미생물 군집의 종 다양성 및 균등도를 확인하기 위해 Shannon index 및 Inverse Simpson index를 구하고, Chao1 값을 통해 Alpha diversity 정보를 확인하였다. 사료 내 미세조류 첨가 효과에 따른 장내 미생물 변화는 각 처리군과 대조군 간 유의적 차이 분석(P<0.05)을 위한 Linear discriminant analysis (LefSe)(Segata et al., 2011)를 실행하여 3 이상의 LDA score를 effect size로 하여 지표로써 사용하였다.
결과 및 고찰
사료 내 미세조류(TO) 첨가가 장내 선천성 면역에 미치는 영향을 확인하기 위해 장관 내 고유층의 선천성 면역세포 분석을 실시하였다. 장관 고유층이란 점막 상피의 얇은 결합조직 층으로 동물 체내 80~90%의 면역세포가 존재하고 있으며 T 세포, 항원제시세포 등 다양한 면역세포가 존재하는 부분이기 때문에 장관 고유층에서 유세포 분석을 실시하였다. 우선 선천성 면역세포 중 면역반응의 중요 조절 세포인 항원제시세포(antigen presenting cell, APC)의 분포를 비교하였다(Fig. 1). 항원제시세포는 MHC II 도움을 통해 항원을 세포 표면에서 제시한다. 이를 통해 T 세포가 침입한 항원을 인지하도록 하여 세포성 면역반응을 유도하는 중요한 역할을 담당하고 있다(Weaver and Unanue, 1990). 항원제시세포를 분석하기 위해 항원제시세포의 대표적 바이오마커인 MHC II를 이용하여 소장 내 항원제시세포의 분포를 확인한 결과 육계 사료 내 미세조류 첨가는 유의한 차이를 나타내지 않았다(Fig. 1A). 항원제시세포는 크게 대식세포, 수지상세포, B 세포로 구성되어 있으므로 비록 미세조류 첨가가 전체 항원제시세포의 분포에는 영향을 주지 않았지만 우리는 미세조류 첨가가 항원제시세포를 구성하는 세포들에 미치는 영향을 추가적으로 분석하였다. 항원제시세포 (MHCII+ cells) 중 Mono/Mac을 발현하는 MHCII+Mono/Mac+ cell을 선별하여 대식세포 분포를 확인하였다. 닭의 대식세포는 식균작용, 항원제시, 사이토카인 생산과 같은 선천성 면역계의 중요한 역할을 담당하고 있다. TLR(toll-like receptors)을 사용하여 병원성 박테리아 및 바이러스에서 유래하는 병원체 관련 분자 패턴을 인식하여 염증 반응을 시작하고 TLR 신호 전달 경로를 활성화하는 것으로 알려져 있다(Barjesteh et al., 2014). 또한 IFN-1, 염증성 사이토카인, 항염증성 사이토카인 및 케모카인 발현을 유도한다(Hong et al., 2021). 본 실험에서 미세조류 첨가는 대식세포의 분포를 감소시키는 경향을 보였으나 대조군 그룹 내 해당세포의 편차가 커서 유의적인 차이를 나타내지는 않았다(Fig. 1B). 분석 시, MHCII+Mono/Mac-cell 또한 분석하였는데 해당 세포 군집에는 수지상세포와 B 세포가 포함되며 사료 내 미세조류 첨가효과는 나타나지 않았다(Fig. 1C). 수지상세포는 선천성 면역에서 매우 중요한 역할을 수행하며 닭에서 성숙한 수지상세포는 T 세포를 활성화시켜 바이러스에 대한 면역력을 증가시켜 준다고 알려져 있다(Xiang et al., 2018).
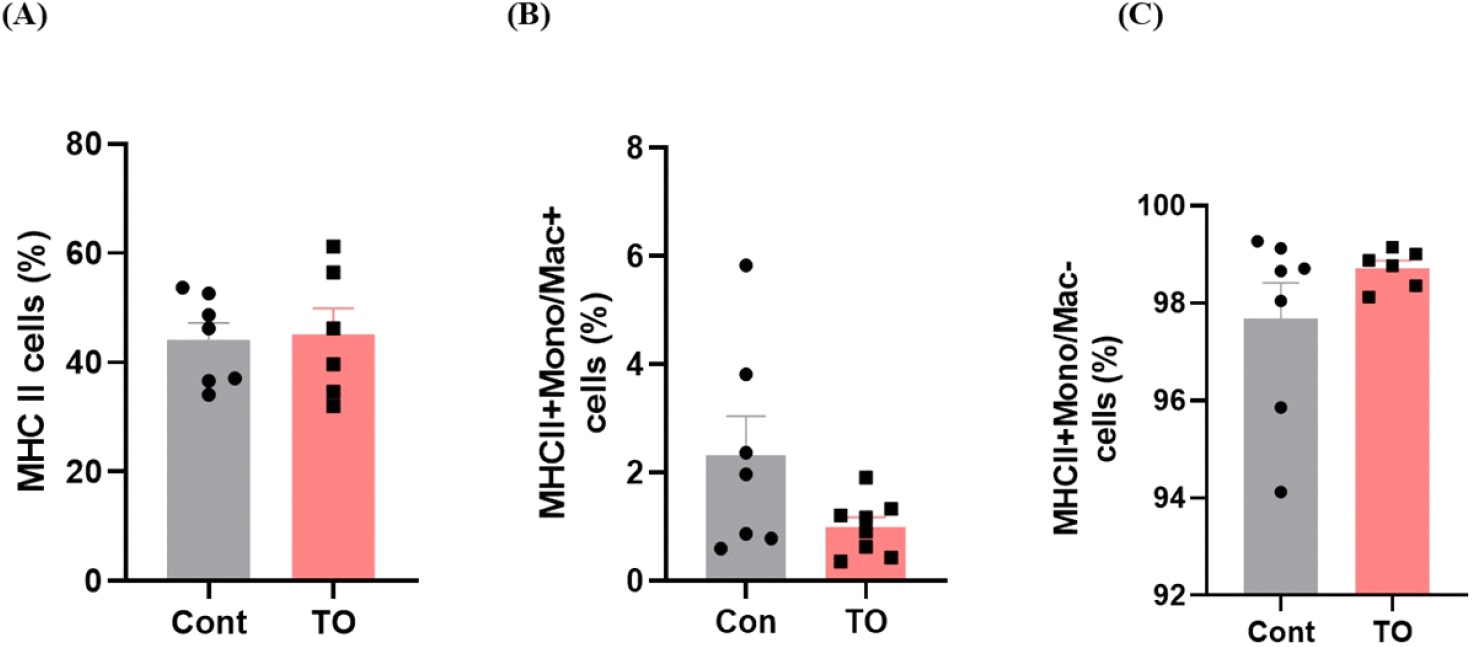
사료 내 미세조류 첨가에 따른 육계 장관 내 B 세포 반응을 좀 더 심도 있게 조사하기 위하여 유세포 분석을 추가적으로 실시하여 소장 내 B 세포의 분포를 조사하였으며, 분변에 존재하는 IgA 농도를 ELISA 분석을 통해 측정하였다(Fig. 2). B 세포는 형질세포로 분화되어 외부로부터 침입하는 항원에 대한 항체를 생성해내는 림프구이며 일부 B 세포는 장기간 생존하는 기억세포로 남아서 같은 항원에 다시 노출되었을 때 더욱 빠른 면역 반응을 가능하게 한다(Bonilla and Oettgen, 2010). IgA는 장관의 항상성을 유지하는데 중요한 역할을 담당하며 점막층과 소화기, 호흡기 내강에서 유입된 병원체들을 중화하고 세포성 면역이 일어나기 전에 감염의 일차 방어 역할을 담당한다(Macpherson and Slack, 2007).
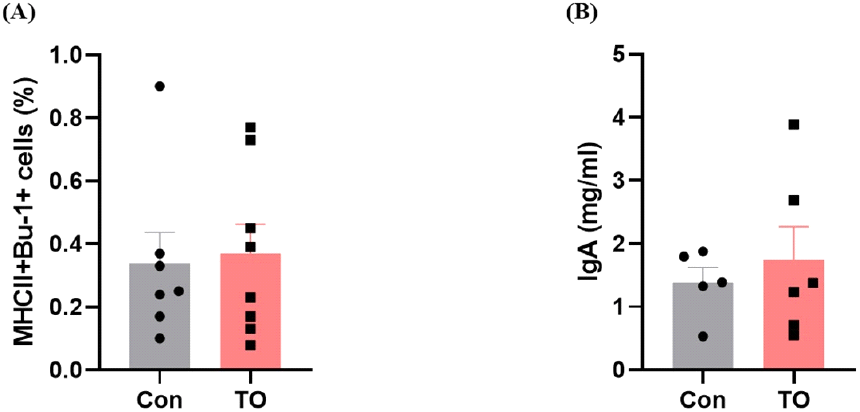
닭 배아의 비장과 골수에 존재하며(Pal et al., 2020), B 세포 마커인 Bu-1을 사용하여 B 세포(Bu-1+MHCII+cell)의 분포를 확인한 결과 사료 내 미세조류 첨가 시 유의한 차이를 나타내지는 않았다(Fig. 2A). 또한, 분변 내 IgA 농도도 대조군 그룹과 미세조류 첨가 그룹에서 유의한 차이를 나타내지 않았다(Fig. 2B).
사료 내 미세조류 첨가에 따른 육계 소장 내 T 세포의 분포를 유세포 분석 기법을 이용하여 비교하였다(Fig. 3). 전체 T 세포의 바이오 마커인 CD3를 이용하여 육계 소장 내 전체 T 세포의 분포를 확인한 결과 사료 내 미세조류 첨가 효과는 관찰되지 않았다(Fig. 3A). 하지만 흥미롭게도 사료 내 미세조류 첨가가 소장 고유층 내 특정 T 세포 분포에 변화를 유도하는 것을 확인하였다. T 세포는 크게 CD4+ T cell, CD8+ T cell, γδ T cell로 구성되어 있다. 이 중 미세조류 첨가는 CD3+CD4+ T cell의 분포를 증가시키고(Fig. 3B, P<0.001), CD3+CD8+ T cell의 분포는 감소시키는 것을 확인하였다(Fig. 3C, P<0.05). CD4+ T cell 분포가 증가하는 결과는 사료 첨가제로써 프로바이오틱스를 급여하였을 때, CD4+ T cell이 증가하였다는 Kwak et al. (2011)의 연구결과와 일치하였다. CD4+ T cell은 다양한 사이토카인 분비를 통해 전체적인 세포성 면역반응 조절에 중요한 역할을 담당하는 면역세포로 알려져 있다. 예를 들어 CD4+ T cell은 CD8+T cell과 B cell을 도와 바이러스에 감염된 세포들을 죽이는데 기여한다(Swain et al., 2012). 또한 신생 동물의 흉선 및 비장과 같은 말초 면역 기관에서 CD4+ T cell이 증가하면 닭의 면역력을 높여준다고 알려져 있으며, CD4+ T cell은 바이러스 감염으로부터 저항성을 갖는데 중요한 역할을 한다(Dai et al., 2019). 또한 일부 CD4+ T cell은 바이러스를 직접 죽이기도 하며(DiPiazza et al., 2016), 닭에서도 이러한 CD4+ T cell의 역할에 대한 연구가 많이 보고된 바 있다(Rauf et al., 2011). CD8+ T cell은 바이러스 침입에 대한 면역력과 관련되어 있다. 일반적으로 CD4+ T cell과 CD8+ T cell의 분포는 역의 상관관계를 가지며 변화된다고 알려져 특정 스트레스 반응이나 질환을 진단하기 위해 CD4+ T cell:CD8+ T cell ratio를 바이오 마커로써 사용하기도 한다(Inoue et al., 2021). 유의미한 차이가 없었던 전체 T 세포 분포에 비해 사료 내 미세조류를 처리한 그룹에서 CD4+ T cell 양의 증가는 상대적으로 CD8+ T cell의 분포 감소를 유도한 것으로 보인다. γδ+ T cell은 αβ T-수용체를 발현하는 대부분의 T 세포와 달리 γδ T-수용체를 발현하며 장내 mucous에 많이 분포한다(Komori et al., 2006). 닭의 비장세포 및 장세포에서 γδ+T 세포는 세포독성을 유발한다고 보고되었다(Fenzl et al., 2017). CD3+CD8+γδTCR+ cell의 분포는 유의한 차이가 존재하지 않았고(Fig. 3D), CD3+CD8-γδTCR+ cell의 분포는 사료에 미세조류를 첨가해주었을 때 감소하는 경향을 보였다(Fig. 3E). 미세조류 첨가에 의한 T 세포 변화에 따른 장관면역 변화가 닭의 전체적인 면역성상에 어떠한 역할을 담당하는지 이해하기 위해서는 감염 모델을 이용한 동물실험이 후속 연구로써 필요할 것으로 보인다.
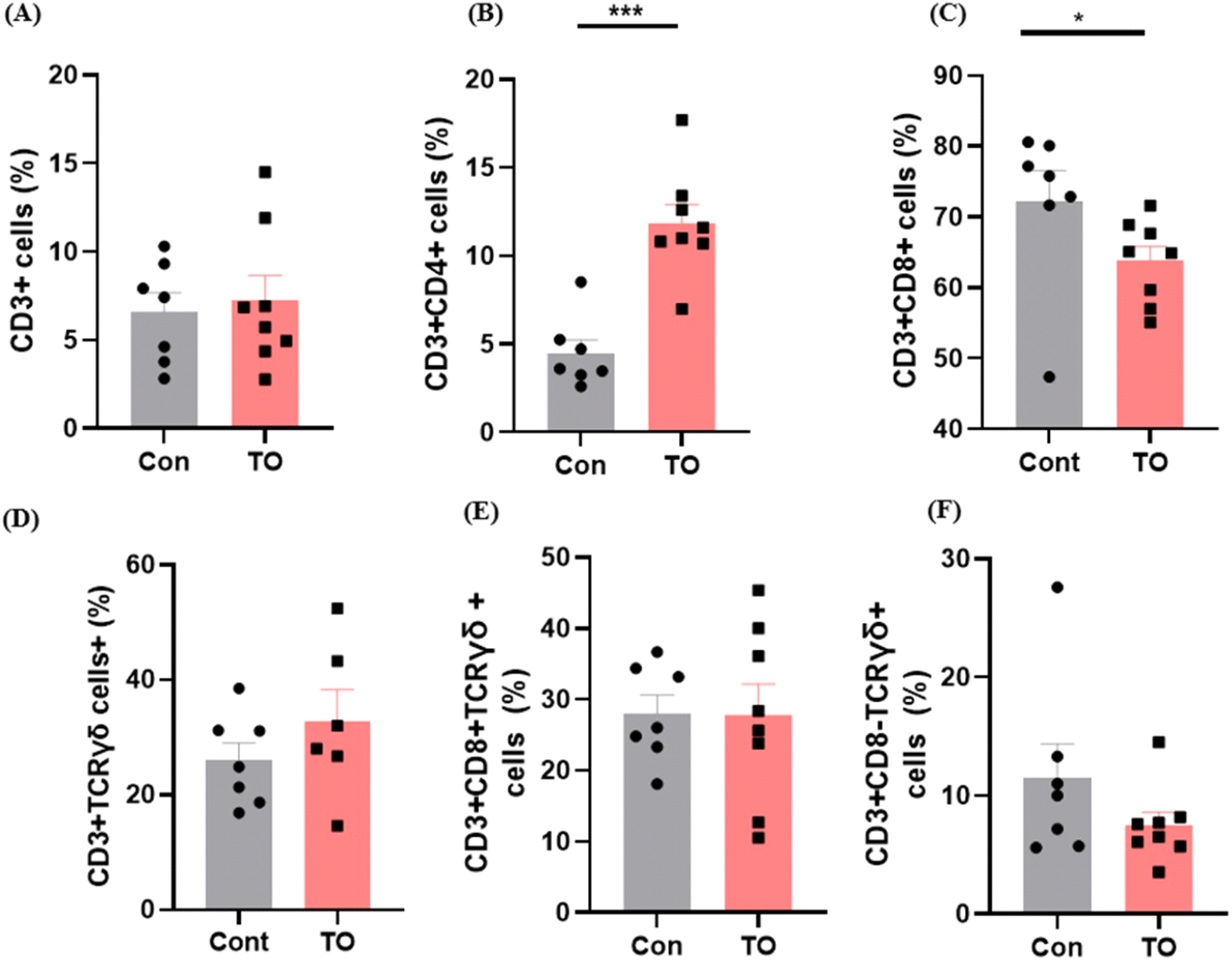
닭의 소화장관은 영양대사 조절 및 면역체계 강화를 통해 닭의 성장과 건강에 중요한 역할을 하는 장내 미생물 집단을 가지고 있다(Diaz Carrasco et al., 2019; Stanley et al., 2013). 닭의 장내 미생물은 주로 phylum 수준에서 Firmicutes, Bacteroidetes, Proteobacteria, Actinobacteria가 차지하고 있다(Diaz Carrasco et al., 2019; Clavijo and Flórez, 2018). 소화 장관은 동물 체내에서 공생 미생물이 가장 많이 분포하는 곳으로, 장내 미생물은 병원균 침입 후 군집 형성을 방지하는 기전으로 작동할 뿐 아니라 면역의 발달과 성숙을 조절하여 동물의 건강상태에 많은 영향을 미친다(Borda-Molina et al., 2018). 또한, 장내 미생물은 다양한 소화효소 분비를 통해 미생물 대사산물을 제공하여 숙주에 새로운 형태의 영양분을 공급하기 때문에 장내에 다양하고 풍부한 미생물의 분포가 숙주에 긍정적인 영향을 미친다는 것이 보고되고 있다(Gaggìa et al., 2010; Roberts et al., 2015; Sergeant et al., 2014). 본 실험에서 TO 첨가에 따른 장내 미생물 군집에 대한 다양성과 풍부도 변화를 조사를 위하여 ASVs, Chao1, Shannon, Inverse Simpson index를 사용하여 분석을 실시하였다(Fig. 4). 분석결과 대조군에 비해 미세조류를 첨가한 그룹에서 종 풍부도를 나타내는 ASVs number와 Chao1 index가 유의적으로 높은 것을 확인하였다(Figs. 4A and 4B, P<0.05). 반면, 종의 다양성을 나타내는 Shannon index와 Inverse Simpson index 비교에서 실험군 간 유의적인 차이는 없었다(Figs. 4C and 4D). 이 결과는 Kwak M-J et al. (2021)에서 육계의 사료 내 생물계면활성제인 sophorolipid를 첨가하였을 때, 맹장 내 분변에서 미생물 군집을 확인한 결과와 일치하였다. 장내 미생물의 종 풍부도가 낮은 가금류는 영양소 흡수가 원활하지 않았고, 이로 인해 성장률이 감소하는 원인이 될 수 있다고 보고하였다(Bailey, 2010). 따라서 종 풍부도가 높은 장내 미생물을 가진 육계 생산은 생산성 향상과 연관되어 있을 것으로 예상된다.
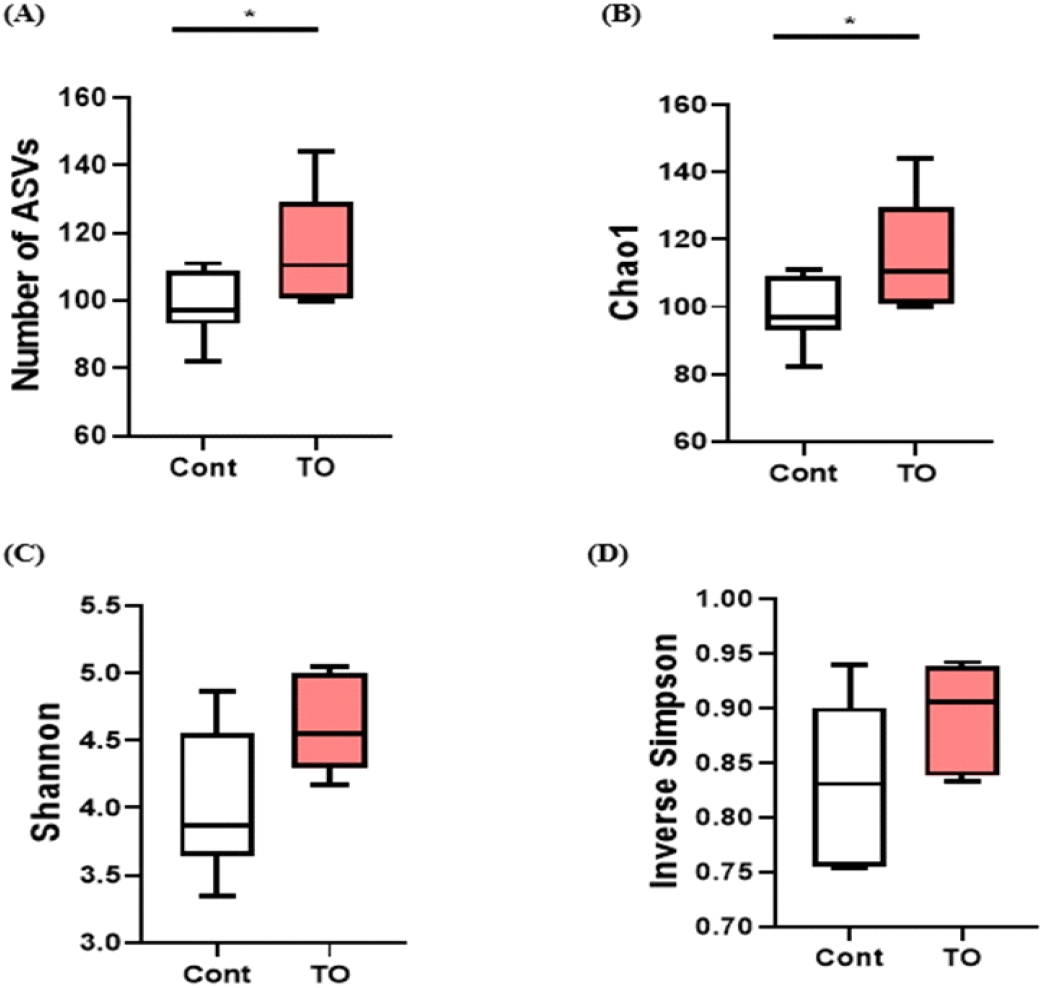
미세조류를 첨가한 초기 육계 맹장 내 분변의 균총 분포도 변화는 Miseq 데이터베이스를 기준으로 분석하였다(Fig. 5). 생물학적 분류 단계 중 Phylum(문) 단계에서 초기 육계의 맹장 내 분변의 균총 분석 결과에서 육계의 분변 내 우세종 순서로 Firmicutes, Bacteroidetes, Proteobacteria, Actinobacteria 순으로 나타났으며, 이는 닭의 맹장에서 확인한 Wei et al. (2013) 결과와 일치하였다. 그룹과 상관없이 Firmicutes과 Bacteroidetes이 90% 이상을 차지하였으며, 주로 병원성균이 많이 속해 있는 Proteobacteria의 경우 미세조류 첨가 시 감소하는 경향을 나타내었다(Fig. 5A).
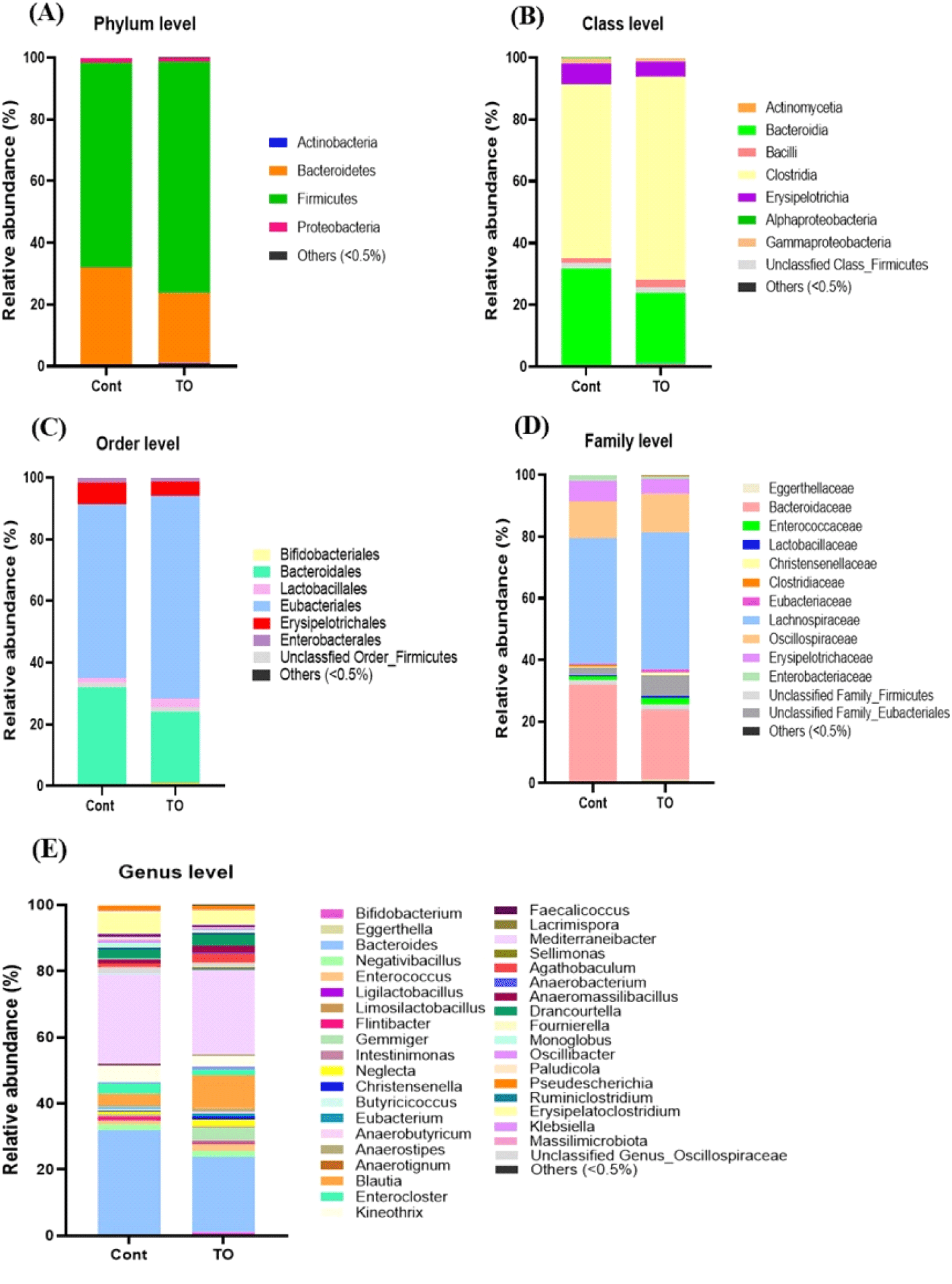
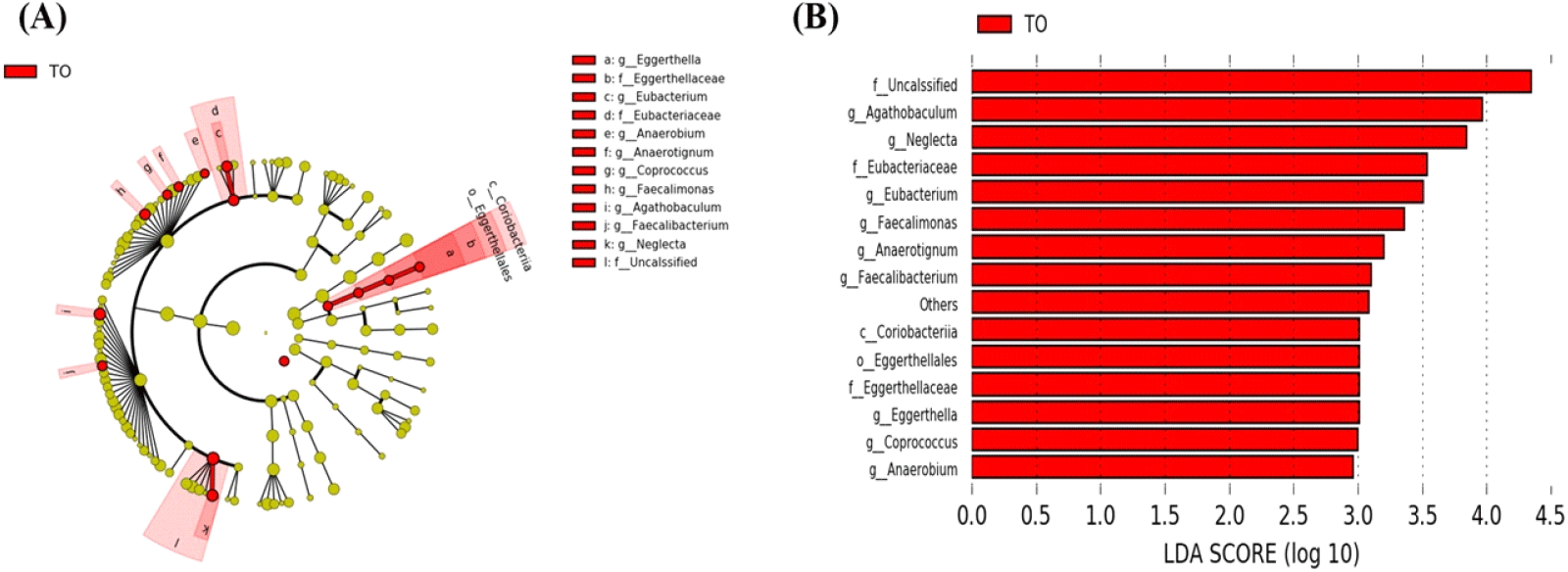
한편, Class(강) 단계에서 분변 내 균총분포도를 분석한 결과, 처리 그룹에 상관없이 Clostridia과 Bacterodia이 각각 약 50%, 20% 이상의 풍부도를 나타내어 대조군 그룹보다 높은 경향을 나타내었다(Fig. 5B).
생물학적 분류 단계 중 Order(목) 단계에서 균총을 분석한 결과, Bacteroidales, Eubacteriales, Erysipelotrichales이 주요 Order(목)로 존재하고 있음을 알 수 있었다(Fig. 5C). 유익균을 많이 포함하는 Lactobacillales의 분포도는 대조군 그룹(1.38%)에 비하여 미세조류를 첨가한 TO 그룹(2.64%)이 상대적으로 높았다.
Family(과) 단계에서 분변 내 균총분포도를 분석한 결과, 그룹에 상관없이 Bacteroidaceae와 Lachnospiraceae가 약 40% 이상을 차지하였다(Fig. 5D). 또한, 유익균을 많이 포함하는 Lactobacillaceae의 분포도는 미세조류 첨가 그룹에서 0.61%로 대조군 그룹의 0.27%에 비해 높은 경향을 나타내었고, 유해균을 많이 포함하는 Enterobacteriaceae의 분포도는 미세조류 처리 그룹에서 수치적으로 낮은 경향을 나타내었다. Enterobacteiaceae는 항생제 내성 효소인 extended-spectrum beta-lactamases(ESBL)를 생성하는 장내 세균으로 알려져 있으며, 인간과 육계의 사이에서 내성균의 전파를 조사하기 위해 연구된 바 있다(Saliu et al., 2017; Projahn et al., 2018).
생물학적 분류 단계 중 Genus(속) 단계에서 균총을 분석한 결과 최소 65개의 미생물 속이 존재함을 확인하였다. 이 중에서 특히 Bacteroides, Blautia, Negativibacillius, Enterocloster, Kineothrix, Mediterraneibacter, Erysipelatoclostridium은 1.0% 이상을 차지하여, 초기 육계 장내 주요한 속임을 알 수 있었다. 각 그룹별로 비교한 결과, 대조군 그룹에서는 Bacteroides가, TO 그룹에서는 Blautia가 다른 그룹에 비하여 높은 빈도를 나타내었다(Fig. 5E).
초기 육계에 미세조류 첨가 시 장내 미생물의 분포 중 통계적 유의성을 가지는 균을 발굴하기 위하여 LEfSe 분석을 실시하였다. 이를 위해 분석 시 P<0.05, LDA score가 3이상인 OTU를 선별하였다. 분석 결과 미세조류 첨가 시 몇몇 장내 미생물의 빈도가 증가하는 것이 관찰되었다. 미세조류 첨가 그룹에서 Coriobacteriia class (Eggerthella, Eggerthellaceae, Eggerthellales), Eubacterium family (Eubacteriaceae), Faecalibacterium, Agathobaculum이 유의적으로 높은 수준으로 존재하였다(Figs. 6A and 6B, P<0.05). 닭의 장내에서 Faecalibacterium의 기능은 명확히 밝혀지지 않았으나, Liu et al. (2018)은 Salmonella enteritidis에 감염된 닭의 장내 미생물 구성에서 Faecalibacterium이 감소하였고, 이는 프로바이오틱 특징을 가지는 미생물 후보로써 사용될 수 있음을 시사한다고 보고하였다(Khan and Chousalkar, 2020). TO 그룹에서 증가한 Agathobaculum은 butyrate 생산에 효과가 있다고 보고된 바 있다(Ahn et al., 2016). 단쇄지방산 종류 중 하나인 butyrate는 장관에서 중요한 에너지원으로 성장촉진, 항균 활성, 면역 조절 및 항염 활성의 역할을 하여 병원체를 감소시킨다고 알려져 있다(Bedford and Gong, 2018).