INTRODUCTION
The broiler chicken industry is one of the foremost and fast-growing active sub-sector in the world agribusiness that pampers global protein nutrition. The consumer perception towards health benefits of chicken meat, low price, convenience in preparation and low religious constraints make the consumption of chicken more popular around the world (Pouta et al., 2010; Wideman et al., 2016; Attia et al., 2017; Petracci et al., 2019). World broiler meat production has been estimated at approximately 90 million tons/year from 21 billion broiler chickens where broiler meat is the dominant meat source that covers 89% of the total poultry meat production in the world (Blake and Tomley, 2014). It has been forecasted that higher consumer demand for meat products and the accelerated increment of the global human population (the current population of 7.6 billion expected to increase to 9.8 billion by 2050) will increase the global meat consumption exponentially from 330 to 455 million tons per year by 2050, while broiler chicken will be covered 40% of the increased demand (Fatoba and Adeleke, 2018; Izar-Tenorio et al., 2020). The United State of America is the world’s largest broiler meat producer (20% of global output), annually rear 9 billion broiler chickens and a consequence of USD 65 billion in revenue, then followed by China, Brazil and the European Union respectively (FAO, 2018; Izar-Tenorio et al., 2020). Also, the better feed efficiency of chickens and relatively lower cost of production make broiler meat production more beneficial and more effective even in developing countries that have limited agriculture resources (Chang, 2007).
Broiler health is a vital factor that affects the growth and global competition of the poultry industry along with the cost of production in the present, and also the strategic future of the industry (Hafez and Attia, 2020). In this regard, infectious diseases from several pathogens such as viruses, bacteria, parasites and fungi either as an individual basis (mono-causal) or in combination with different other microorganisms (multi-causal) could hamper the health and productivity of broilers, thus increased mortality (Fatoba and Adeleke, 2018; Hafez and Attia, 2020). Furthermore, infectious diseases may lead to substantial economic losses through reduced revenues, reduced quantity or quality of meat, and increased costs of medications and labour along with affecting food security and risk to public health through zoonoses (Wubet et al., 2019; Asfaw et al., 2021). Parasitic diseases are proven to cause the most adverse effects namely higher mortality, impaired growth, worsen feed efficiency and anemia of broilers than other bacterial or viral diseases (Ruff, 1999; Kaufman et al., 2007). Besides, the study of Nnadi and George (2010) is well documented that immunosuppression (especially in terms of vaccination) could be developed due to the parasitic infections in broilers.
A living organism that depends on another organism (the host) for nourishment, shelter, protection and reproduction is defined as a parasite and the dynamic association between a host and a parasite is called parasitism (El-Tonsy, 2012; Poulin and Morand, 2014). As a consequence, pathogenic parasites lead to having poor clinical manifestations such as mechanical injuries, impact effects from toxic compounds, deprivation of nutrients and immunosuppression of the host (Taliaferro, 2009; Garcia et al., 2018). Parasites in broiler chickens can be classified into three major groups based on their inhibition as follows (Permin and Hansen, 1998; Table 1):
Coccidiosis is the most catastrophic and commonly encountered parasitic disease in the poultry and the infection by parasites (coccidia) in an adequate number to produce clinical manifestations of the disease is called coccidiosis (Conway and McKenzie, 2007; Sharman et al., 2010; Lawal et al., 2016; Ali et al., 2019; Kim et al., 2019). Poultry coccidiosis has been studied for over a century and the first identification of coccidiosis as a parasitic disease by several species of Eimeria was published in 1910 (Reid, 1990). In the late 1920s and early 1930s, fundamental studies were initiated to study the life cycle and pathogenicity of Eimeria spp. and invent vaccines and chemical medications to control coccidiosis. The USA has introduced prophylactic ionophores antibiotics and started a study to invent a safe vaccine (launched 20 years later) during the 1970s (Shirley and Lillehoj, 2012).
This enteric disease can be found globally in all segments of the poultry industry (i.e., broilers, layers, breeders) and it has been estimated of exceeding USD 3 billion economic impact, annually, due to the production losses and cost for prevention, nutrition, and medications (Blake and Tomley, 2014; Shivaramaiah et al., 2014). Eimeria infection intimidates broiler health and welfare by invading the gut epithelial cells and causes tissue damages (enteritis), interruption to the digestion process and nutrient absorption thus poor feed efficiency (Yegani and Korver, 2008; Soutter et al., 2020). Moreover, coccidiosis leads infected broilers to have bloody diarrhoea, delayed sexual maturity, and eventually higher morbidity and mortality rates (especially young broilers; the age of 3-18 weeks) (Dakpogan and Salifou, 2013; Abdisa et al., 2019).
ETIOLOGY OF COCCIDIOSIS IN CHICKENS
Coccidiosis in chickens is caused by intracellular apicomplexan protozoan parasites belonging to the genus Eimeria and are frequently vulnerable to seven species of coccidia (Conway and McKenzie, 2007; Fig. 1). All the Eimeria spp. are host-specific (ubiquitous) and each species infects a certain site of the intestinal tract of chickens with different levels of pathogenicity (Table 2) (De Gussem, 2007; Abdisa et al., 2019). As has been previously reported in the literature of Hadipour et al. (2011) and Gharekhani et al. (2014), coccidiosis can be only transpired in an individual host after the ingestion of sporulated oocysts of Eimeria spp. that have been shed by either clinically infected or recovered birds through their faeces, which contaminate feed, water, bedding, and mechanical carriers (i.e., equipment, clothing, insects, farmworkers etc.).
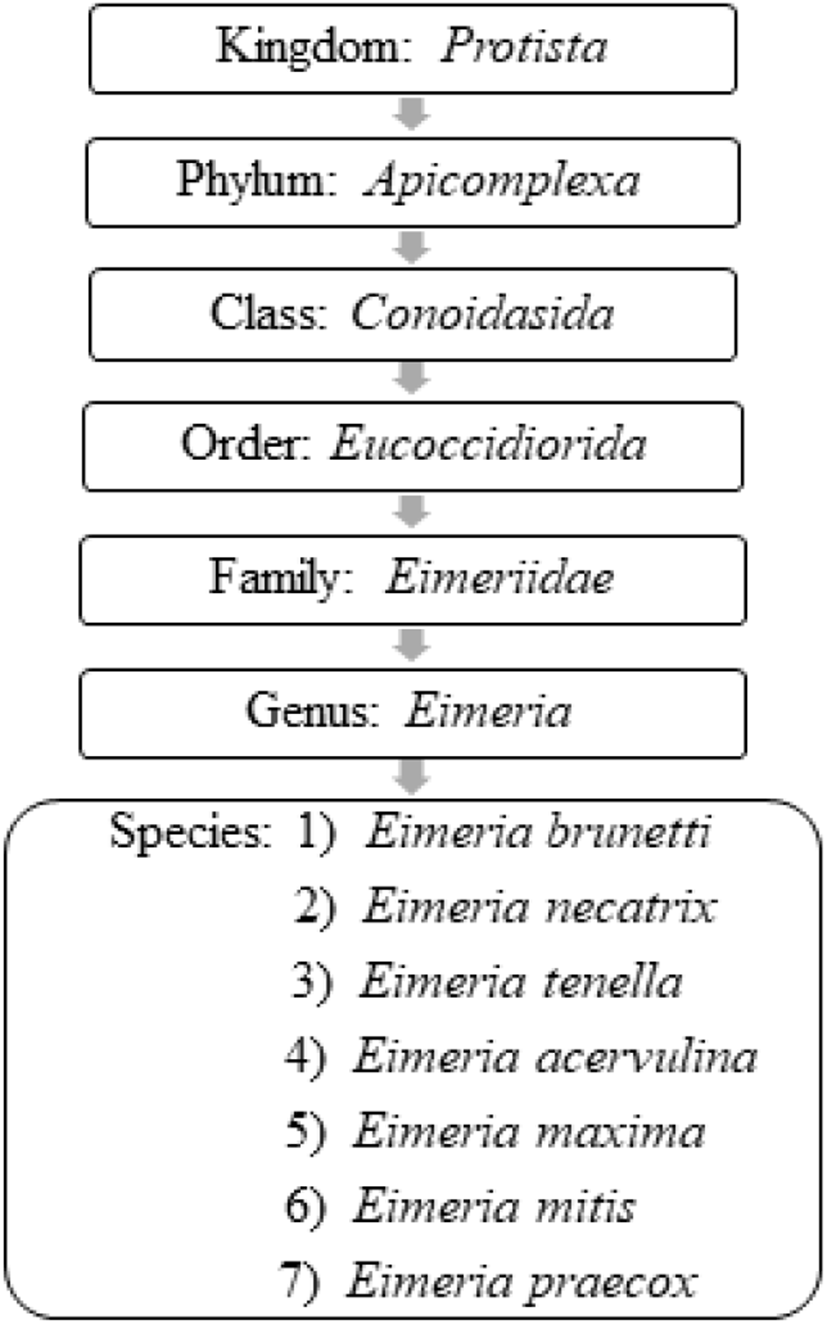
Adapted from Conway and McKenzie, 2007; Quiroz-Castañeda and Dantán-González, 2015.
Oocyst is a solid and robust structure (resistant to mechanical, chemical and proteolytic degradations) made by encapsulating the soft bodies of parasites from a glycoprotein and lipid bilayer wall (oocyst wall) that can be preserved/survived in the outer environment for a prolonged/lengthy-time period even under extreme conditions (Mai et al., 2009; Remmal et al., 2011). Based on the infectious ability there are two types of oocysts (Belli et al., 2006; Lal et al., 2009):
The sporulated oocyst can be survived approximately for 50 months in the external environment (i.e., faeces, litter, feed, soil) until entering a host, whereas, unsporulated oocysts persist for 7 months in the host caecum (Blake and Tomley, 2014; Fatoba and Adeleke, 2018). Unsporulated oocysts can be converted into sporulated oocysts with the presence of favourable conditions (i.e., O2, moisture and warmth) and this process is known as sporulation (Canning, 1968; Quiroz-Castañeda and Dantán-González, 2015). Sporulated Eimeria oocyst (Fig. 2) is comprised of four sporocysts and each of them containing two convex/banana- shaped sporozoites which are considered as the infectious stage (Belli et al., 2006; López-Osorio et al., 2020). These oocysts are shed through the faecal materials from infected birds and the coccidia infection can occur after the ingestion of oocysts by a susceptible chicken (Gharekhani et al., 2014; Shivaramaiah et al., 2014). The amount of ingested sporulated oocysts determine the severity of the intestinal damage of the bird (Charlton et al., 2006; Fatoba and Adeleke, 2018).
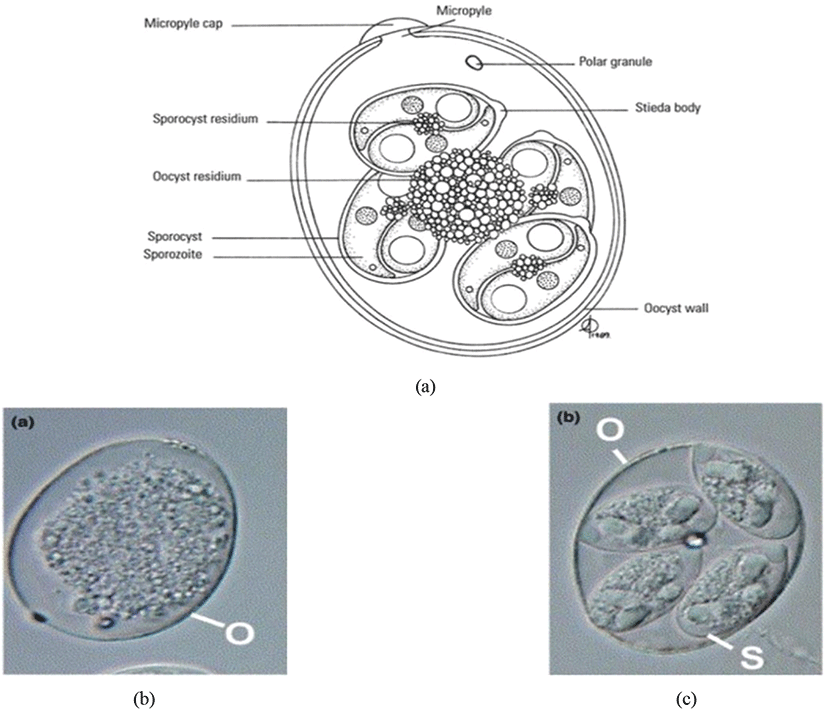
The life cycle of an organism is defined as the sequence of sudden developmental (maturation) changes in the morphology and/or ecology of that particular organism (Benesh, 2016). Eimeria spp. have a homoxenous complex life cycle (Fig. 3) that comprises of two developmental stages namely the exogenous phase (occurs in the outer environment; sporogony) and the endogenous phase (occurs in the intestine of the host; schizogony and gametogony) (Allen and Fetterer, 2002; López-Osorio et al., 2020).
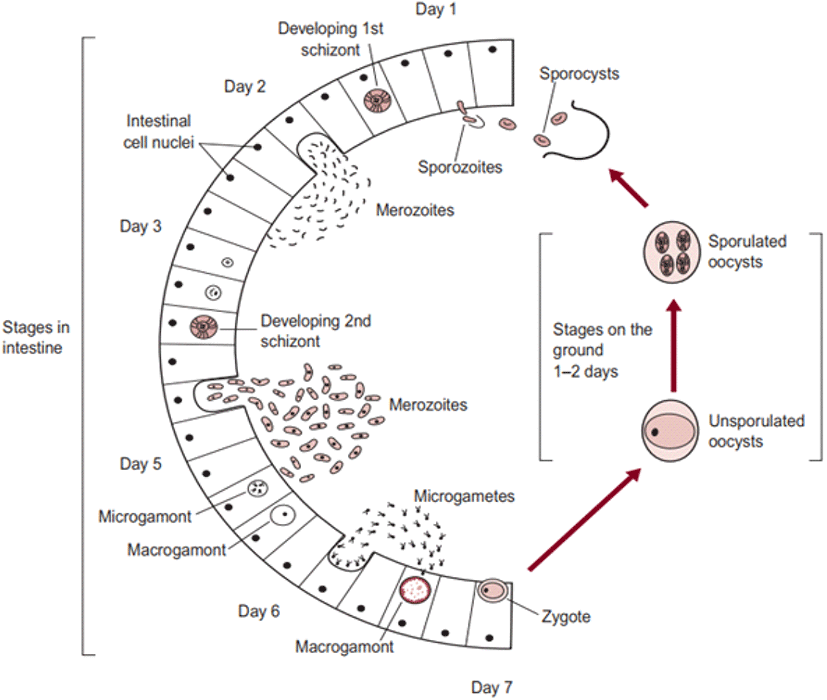
In the exogenous phase, unsporulated oocysts get sporulated by completing miosis and mitosis to produce haploid sprozoites under favourable conditions within 24-48 hours (Shirley and Harvey, 1996; Pattison et al., 2007). The mechanical and chemical (i.e., trypsin, bile salt, carbon dioxide) degradation of the gut liberates the sporozoites (Fig. 4) and they invade the epithelial cells in a specific site of the intestine according to the species involved. After the invasion, sporozoites converts into a feeding stage named trophozoites (12-48 hours), trophozoites get enlarged and asexually multiply/divide the parasite nucleus to complete the schizogony phase (Conway and McKenzie, 2007; Pattison et al., 2007). During a couple of schizogony cycles (number of cycles determined genetically depending on the species) trophozoites generate a large number of merozoites that infect the new cells in both the small and large intestine (Allen and Fetterer, 2002; Blake et al., 2020). Matured merozoites undergo a single round of sexual gametogony phase that resulting in the formation of male microgametes and female macrogametes, and both of these gametes (Fig. 5) are responsible for the clinical signs of coccidiosis such as lesions, imbalance nutrient absorption and diarrhoea (Levine, 1973; Deplazes et al., 2016). Finally, microgametes are surrounded by macrogametes (fertilize), developing the oocysts and are released into the faeces through ruptures of epithelial cells (Deplazes et al., 2016; López-Osorio et al., 2020).
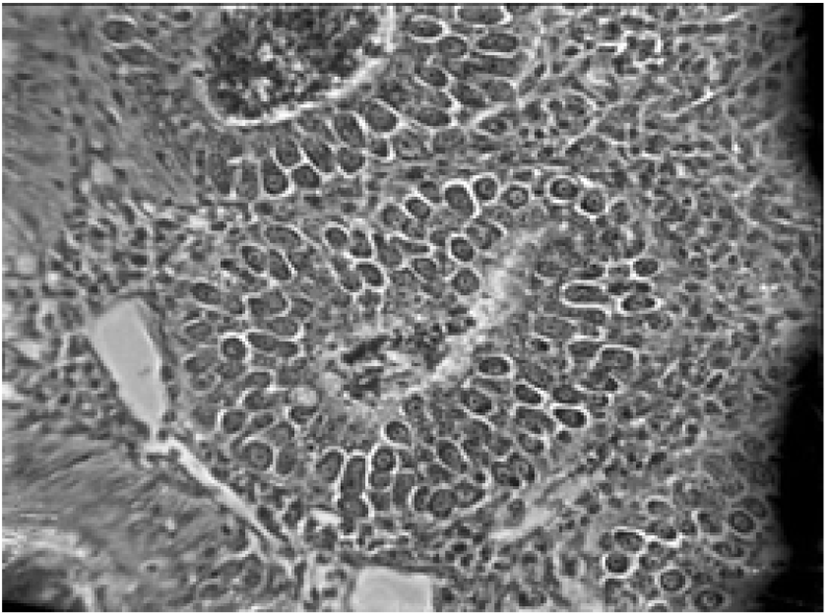
Pathogenicity aggravates with the increasing number of sporulated oocysts ingested by the host and the species of the Eimeria (see Table 2) (Morris and Gasser, 2006; Répérant et al., 2012). Eimeria infection extensively destructs the intestinal epithelial cells and tissues, thus suppresses the gut stability of chickens (Chen et al., 2020). Consequently, it may lead to impaired digestion of feed and nutrient absorption of chickens, altered serum protein and electrolyte levels with disturbed ion and osmotic balance of gut epithelium (Fitz-Coy and Edgar, 1992). Moreover, it has been found that the Eimeria spp. change the overall structure of the intestinal microbiota and facilitates the growth of other pathogenic bacteria species (i.e., Clostridium perfringens, Salmonella enterica and Campylobacter jejuni) in the gut due to the tissue damages, thus it will impair the gut health and morphology of chickens (Collier et al., 2008; Macdonald et al., 2019). Besides, coccidiosis reduces the caecal microbial diversity, thus alter the production of short-chained fatty acid in the caeca of the chicken (Stanley et al., 2014). The immunity suppression caused by other diseases may exacerbate coccidiosis conditions of birds, for instance, Marek’s disease elevates the severity of coccidiosis in broilers (Abebe and Gugsa, 2018).
Coccidiosis of chickens can occur in two forms as sub-clinical coccidiosis and clinical coccidiosis (Abebe and Gugsa, 2018). Worsen feed efficiency, reduced growth rate, and ultimately higher mortality and morbidity rates can be observed in sub-clinical coccidiosis (Dakpogan and Salifou, 2013; Abdisa et al., 2019). Chickens with clinical coccidiosis exhibit drooping eyelids/somnolence, ruffled feathers, pale colour comb, wattles and internal organs (due to the blood losses), reduced head size, loss of yellow colour in shanks and beak, catarrhal inflammation and dehydration along with haemorrhagic faeces (Graat et al., 1996; Simon, 2005). The study of Singla and Gupta (2012) has reported that some clinical signs of coccidiosis can be confused with other diseases in chickens, therefore further diagnosis methods are required to confirm the coccidiosis in chickens. (Fig. 6, 7, 8)
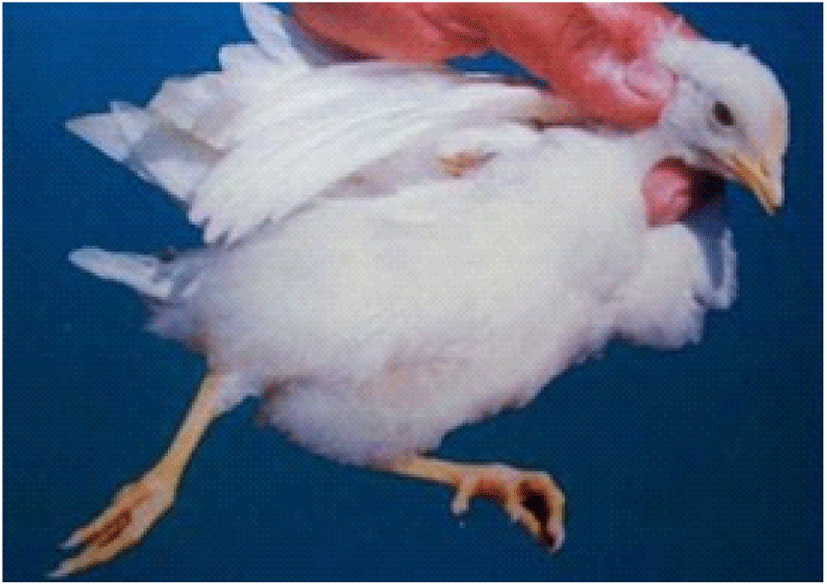
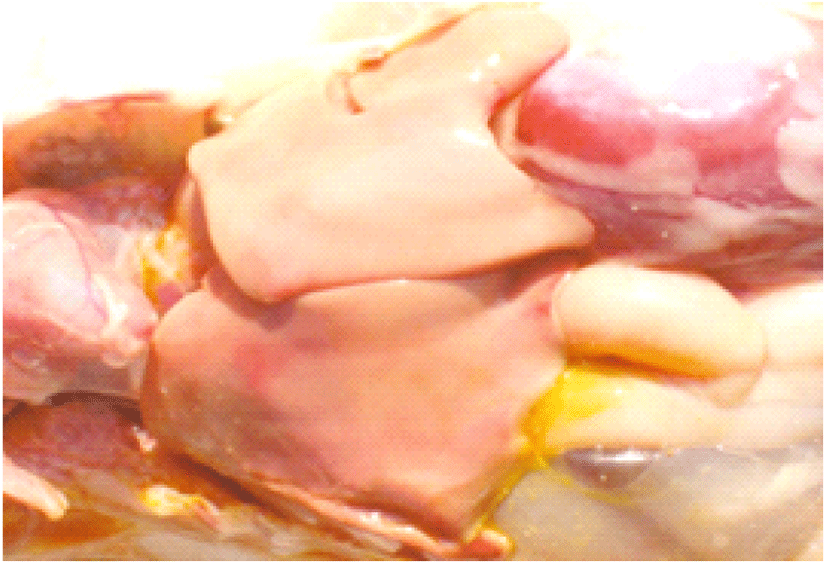
DIAGNOSTIC METHODS FOR COCCIDIOSIS
Joyce Johnson and W. Malcolm Reid have developed a post-mortem examination scoring system which ranked from 0 to +4 (i.e., 0 = no lesion, +1 = mild lesion, +2 = moderate lesion, +3 = severe lesion, +4 = extremely severe lesion) to interpret the severity of poultry coccidiosis based on macroscopically visible lesions in the gut caused by Eimeria spp. (Table 3; Fig. 9). The responsible Eimeria species can be identified by examining the predilection site (since species are site-specific; see Table 4) of the intestine and the score is being given based on the extent of the gross lesion (Singla and Gupta, 2012). The complete intestine (between gizzard and rectum) should be dissected and carefully observed the mucosal surface and serosal surface for lesions under a bright light source, nonetheless microscopic observations are required to check smears taken from susceptible lesions for Eimeria parasites when lesions are not visible clearly (Conway and McKenzie, 2007). The individual lesions scores obtained from a discrete bird for all Eimeria species are generally summed for a considered number of birds to have a total mean lesion score (De Gussem, 2007). However, this method is extremely time-consuming, labour intensive, subjective and need a higher level of expert knowledge (Shirley et al., 2005). Johnson and Reid’s method is more accurate in diagnosing the coccidiosis in chickens for controlled experimental infections rather than actual farming conditions, where the Eimeria spp. and the dose of oocysts are known (McDougald and Fitz-Coy, 2008).
Adapted from Johnson and Reid, 1970.
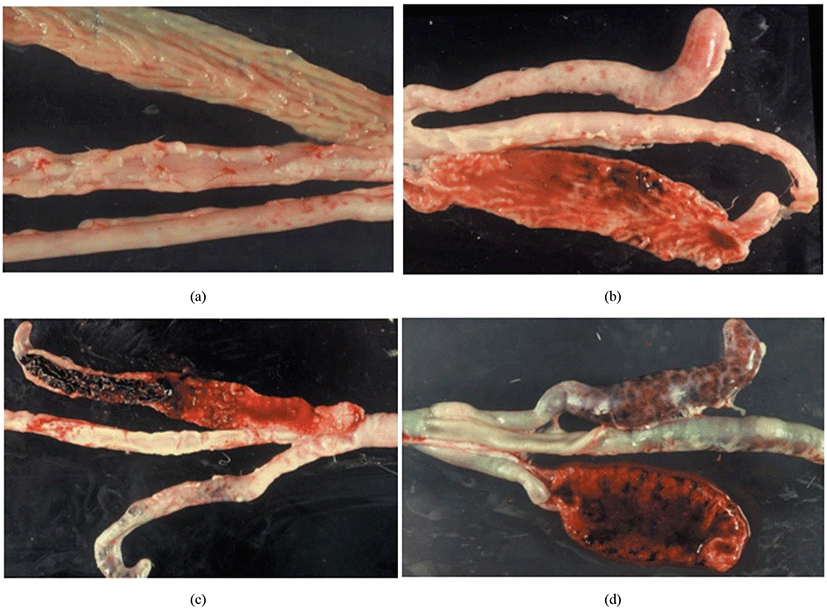
Adapted from Abebe and Gugsa, 2018.
Eimeria oocysts which are excreted through the faeces of infected birds can be detected by faecal examination methods and are the most convenient and inexpensive ways for diagnosing coccidiosis in chickens (Mwale and Masika, 2011). Either way of microscopic observation of oocysts in smears made from emulsified faeces or check the concentration/amount of floated oocysts by floatation technique is the common qualitative faecal examination diagnosing methods for coccidiosis in chickens (Johnson, 1938; Alqomsan, 2010). A solution with a higher specific gravity (i.e., Saturated sodium chloride, 1.19; saturated glucose, 1.44) is used to float the comparatively low specific gravity (1.05-1.15) oocysts to the surface and leave other debris in faeces at the bottom of the solution (Olanrewaju and Agbor, 2014).
A quantitative faecal examination is performed to determine the number of oocysts per gram of faeces (OPG count) along with the percentage of sporulation and oocyst dimensions (Singla and Gupta, 2012). Particularly two methods are followed to enumerate oocysts as follows (Conway and McKenzie, 2007):
McMaster method is the most common laboratory analysis technique for counting Eimeria oocysts in the faecal solution and for determining the individual oocysts shedding pattern of an infected bird (Kaufmann, 1996). The floatation method of oocysts is identical to the quantitative technique and it takes place in a special counting chamber/slide known as the McMaster slide (Fig. 10) to facilitate easy counting through the light microscope (Vadlejch et al., 2011). Notwithstanding, a higher level of expert knowledge is required to distinguish the Eimeria spp. based on their morphology through the McMaster method and OPG count does not elaborate on the negative effect of the Eimeria parasite on the performance of the flock (De Gussem, 2007).
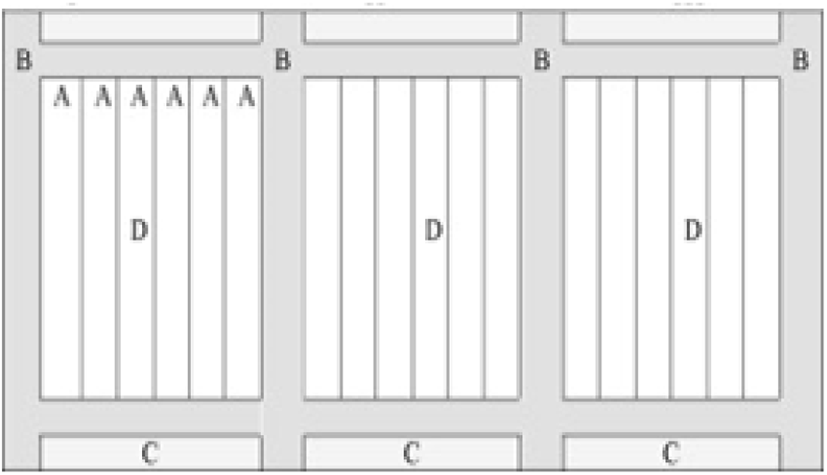
The Hemocytometer method has been used to estimate the cell concentration in a sample that is adopted for computing the coccidia oocysts in faecal solution by using a hemocytometer (Absher, 1973; Conway and McKenzie, 2007). A common preliminary solution preparation method is shared with the McMaster method and the sample being loaded into a special counting slide, Neubauer chamber/ hemocytometer slide (Fig. 11) that consisted of a 0.1 mm deep chamber with a square grid and observe through the light microscope (Arabkhazaeli et al., 2011; Abunemeh, 2016). The Hemocytometer method handily distinguishes Eimeria oocysts, nonetheless, the counting error per millilitre is higher rather than the McMaster method (Conway and McKenzie, 2007).
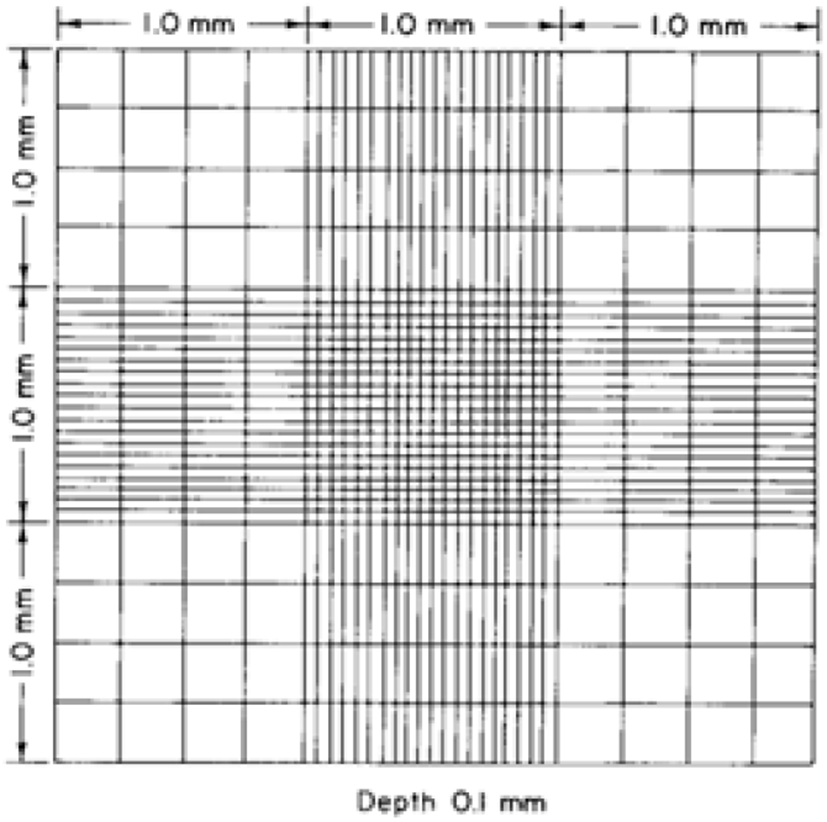
Next-generation laboratory diagnostic methods are indispensable over the aforementioned traditional methods to identify the exact Eimeria spp. responsible for the infection with regards to prevention and control of the coccidiosis (Fatoba and Adeleke, 2018). Besides, the limitations of traditional methods can be overcome by following laboratory- developed diagnostic methods and are species-specific with a higher rate of sensitivity and accuracy (Singla and Gupta, 2012). The enzyme-linked immunosorbent assay (ELISA) is a straightforward and reliable biochemical diagnostic method for coccidiosis that detects epitope-specific antibodies (IgG and IgM) of Eimeria spp. presents in the serum (Smith et al., 1993; Constantinoiu et al., 2007). Moreover, Onaga et al. (1986) reported that ELISA can be used to determine the degree of exposure of Eimeria spp. to a flock and the exposure rate of vaccinated chickens (infectivity in vaccination programs). Even so, results of the ELISA can be impaired due to the cross-reactivity of antibodies that can be observed for multiple Eimeria spp. sporozoites and merozoites in chicken serum (Uchida et al., 1994).
Polymerase chain reaction (PCR) is a highly sensitive molecular diagnostic technique that is used to identify the Eimeria spp. in chickens by examining their variations of genomic DNA (Schnitzler et al., 1998; Alqomsan, 2010). Amplification and identification of excised internal transcribed spacer 1 and 2 (ITS-1 and ITS-2) regions of ribosomal DNA (rDNA) are being practised in PCR, since it has been proven that, the particular region is divergent in between Eimeria spp. and it gives accurate results (Lew et al., 2003; Hamidinejat et al., 2010; Fatoba and Adeleke, 2018). Specific sets of primers and species-specific PCR assays are developed in the present to distinguish five Eimeria spp. namely E. tenella, E. maxima, E. acervulina, E. necatrix, and E. brunetti (Su et al., 2003). Moreover, conserved ribosomal DNA sequences (i.e., 5.8S and 28S) targeting method can be practised to identify the Eimeria spp. in chickens and instead of ITS-1 and ITS-2, Random Amplified Polymorphic DNA (RAPD) is used to develop Sequence Characterized Amplified Region (SCAR) primers for the identification of individual Eimeria species (Fernandez et al., 2003; Cantacessi et al., 2008).
The multi-locus enzyme electrophoresis (MEE) method is followed to individually determine the Eimeria strains in a sample (Morris and Gasser, 2006; Singla and Gupta, 2012). Different strains of Eimeria have unique varieties of enzymes and those enzymes are being separated based on the migration distance through a gel base under the influence of an electric field according to their charge and size (Andrews and Chilton, 1999). By analysing the relative positions of an enzyme, the particular enzyme and the Eimeria spp. who owns that enzyme can be predicted.
Southern blot analysis is also an electrophoresis technique that detects specific/unique fragments of DNA of different Eimeria stains that results from the digestion of genomic DNA by restriction endonucleases (Morris and Gasser, 2006; Singla and Gupta, 2012). Hybridization (using radiolabelled probs) and either autoradiography or fluorography are followed after the electrophoresis to detect the relative position of the species-specific DNA fragments (Ellis, 1990; Morris and Gasser, 2006).
CONTROLLING MEASURES OF COCCIDIOSIS
It is challenging to keep chickens free from coccidiosis due to the omnipresent nature, higher spreading ability and higher reproduction ability of coccidia oocysts (Allen and Fetterer, 2002). Therefore, all the management and biosecurity practices should be targeted to prevent the entry of the coccidia parasites into the farm and/or if the infection already takes place, suppress the multiplication of parasites and the spread of the disease (Peek and Landman, 2011). Cleaning and disinfection of the farm premises between every two flocks are crucial in controlling coccidiosis. Many disinfecting agents such as ammonium hydroxide (both fluid and vapour form; concentration of ≥ 5%), cresol-based products, a combination of formol (37%) and sodium dodecylbenzene sulphonate (12%), and a combination of calcium hydroxide and ammonium sulphate have been identified and used globally for destroying sporulated and non-sporulated oocysts (Peek and Landman, 2011). Due to the possibility of spreading coccidiosis through contaminated litter from excreted oocysts, the litter should be always maintained dry, frequently raked and replace between every two flocks (it suppress the sporulation of oocysts) and also lime powder can be used as a litter drying agent in the rainy seasons (Shivaramaiah et al., 2014; Abdisa et al., 2019). Moreover, separation of the chickens from the external environment, reduction of risky movements in the farm and in between farms, sanitation of the persons and pieces of equipment, proper ventilation, reduction of bird density in the farm and supplementation of clean feed and drinking water can be followed generally to prevent coccidiosis outbreaks in broiler farms (Williams et al., 2000; Peek and Landman, 2011; Quiroz-Castañeda and Dantán-González, 2015).
Chickens are capable of acquiring active immunity and/or passive immunity against poultry coccidiosis to ameliorate its deleterious effects (Lee et al., 2009; Wallach, 2010). The active immunity is provoked by intended infections of either Eimeria oocysts or live parasites (as a mixture of multiple Eimeria spp. and/or strains of virulent or attenuated parasites), which can be persistent and highly specific for the Eimeria spp. (Chapman, 1999; Allen and Fetterer, 2002). Despite the cost and more workforce, vaccination is being identified as the best strategy for controlling coccidiosis in chickens for a long time, which can be practised as follows (Fanatico, 2006; Chapman and Jeffers., 2014; Sokale et al., 2017; Albanese et al., 2018):
-
Spray the vaccine on the feed
-
Mix the vaccine with drinking water
-
Spray cabinet method - Apply through a water-based spray in hatcheries for day-old chicks, ingestion of oocysts can be happened from preening and through their eyes, only 90-95% of chicks will be received the vaccine.
-
Vaccine incorporated edible gel pucks (Fig. 12) – Can be placed inside the house or in transport crates, could be bright in colour to attract chicks, homogeneity of the vaccine in the gel can be less.
-
In-ovo injection (Fig. 13) - Practised for incubated chicken eggs, an individual chick will be received a uniform dose of vaccine and help in developing early immunity against coccidiosis.
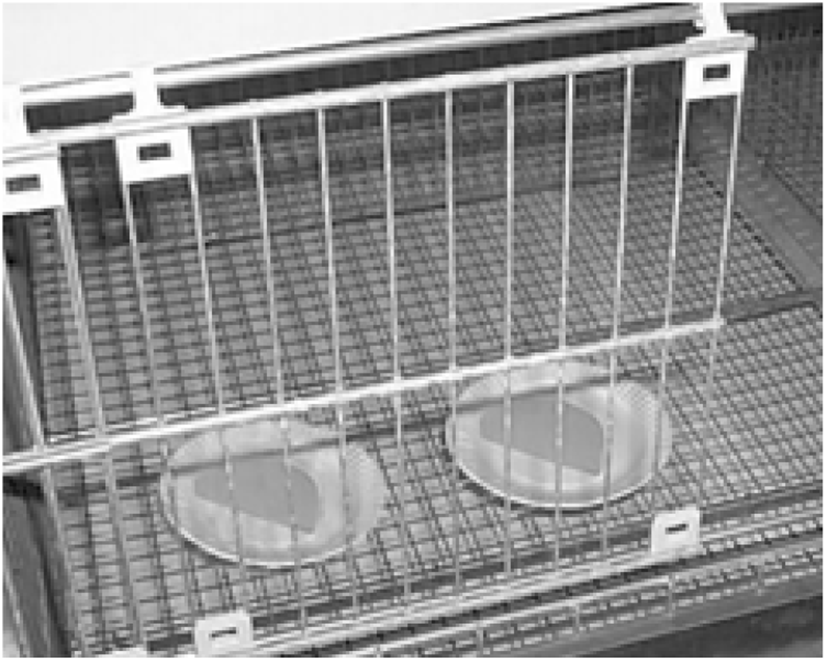
Live vaccines are comprised of either attenuated (precocious) or non-attenuated (virulent/wild-type) Eimeria strains, which help in developing the immunity of birds against coccidiosis by recycling its lower dosage to a progeny (Quiroz-Castañeda and Dantán-González, 2015). Due to the risk of the direct introduction of live parasites to birds, live vaccines have been limited for broiler chickens in the past five decades, nonetheless, with the improved management and administration practises it is applying exponentially in present (Barbour et al., 2015). The use of live vaccines for preventing coccidiosis is upheld by the self-limiting nature of the Eimeria infection, less susceptibleness of younger chicks to the infection (development of immunity can easily occur through oocysts inoculation in the younger stage), and the development of immunity for different Eimeria spp. (McDonald and Shirley, 2009; Price, 2012). At present, live vaccines are proven to be more effective than anticoccidial drugs and there are several kinds of vaccines available in the world market for the sustainable control of coccidiosis in chickens which should be provided to chicks during the first week of age to have strong immunity against coccidiosis by faecal-oral recyclization of parasites (Danforth, 1998; Suo et al., 2006; Soutter et al., 2020). Nevertheless, the high cost of production, gradual decrement of pathogenicity of Eimeria spp. with time, tendency to reverse back to virulence, antigenic diversity, and errors in dosages are identified as major disadvantages of live vaccines (Peek and Landman, 2011).
Attenuated/precocious vaccines are produced by decreasing the virulence of Eimeria spp. strains artificially, either through a serial passage of the parasite in embryonated eggs, irradiation, chemical treatments, or by shortening the endogenous life-cycle of the parasite (precocity) by reducing one or more schizogony stages to produce a lower number of oocysts (Shivaramaiah et al., 2014; Abebe and Gugsa, 2018). Consequently, the lower pathogenic potential of the Eimeria parasites in attenuated vaccines (see Table 5) gives a mild infection and negligible intestinal damages to the bird with an effective immune response and reduced prepatent time (Allen and Fetterer 2002). However, lower fecundity of parasites will increase the cost of production of the attenuated vaccine and sometimes producers are failed to maintain the correct combination of the immunogenicity and attenuation of virulence of the parasites in the vaccine (Price, 2012). Besides, no artificial laboratory or field techniques are practised to alter the natural virulence/pathogenicity nature of Eimeria parasites in non-attenuated vaccines (Williams, 2002; Peek and Landman, 2011). Non-attenuated vaccines (Table 5) provide lifelong protective immunity to the birds against coccidiosis; however, the use is being limited due to the risk accompanied by the direct introduction of wild-type parasites to young birds which causes poor antigenic stimulation in the immune system (Lillehoj and Lillehoj, 2000; Quiroz-Castañeda and Dantán-González, 2015). Moreover, it is necessary to provide the recommended dosage of non-attenuated vaccines to avoid vaccine-induced clinical coccidiosis from the birds (Peek and Landman, 2011).
Adapted from Shivaramaiah et al. (2014) and Peek and Landman (2011).
Instead of providing living parasites or their development stages, recombinant vectored vaccines are consisting immunogenic antigens of Eimeria spp. which are embedded in complementary plasmid vectors to stimulate the immunity of chickens against poultry coccidiosis (Fatoba and Adeleke, 2018; suprihati et al., 2018). These types of vaccines have been considered safe for the host due to the attenuation of the vector organism during the development of the vaccine, and effective than live vaccines due to the lower production cost with higher efficacy (Shivaramaiah et al., 2014; Tan et al., 2017). Subunit vaccines (Table 5) are made out of either purified native gametocyte antigens of virulent Eimeria spp. or from their DNA recombinant proteins in different stages in the life cycle (i.e., sporozoites, merozoites or gametes) which develop humoral and cell-mediated immunity in chickens against coccidiosis infection (Peek and Landman, 2011; Barbour et al., 2015). Generally, the micronemes proteins (MIC) and protein secreted from the apical organelles of the Eimeria parasites (i.e., Apical membrane antigen 1, MITHB, MIC2, MIC3, MIC4 etc.) are considered as the anticoccidial vaccine candidates to produce recombinant vaccines (Shivaramaiah et al., 2014; Blake et al., 2017).
Chemoprophylaxis has been deployed globally as an effective model for the prevention of coccidiosis by the inclusion of a range of anti-coccidial drugs into the poultry diet or drinking water, that provide coccidiostats effect (prevent the replication and growth) and coccidiocidal effect (destruction) upon Eimeria spp. (Chapman and Jeffers., 2014; Quiroz-Castañeda and Dantán-González, 2015). The majority of broad-spectrum anti-coccidial drugs inhibit the asexual phases (first and second), some of them are acting against the sexual phases of the Eimeria life cycle, and the least number of drugs hamper the chemical metabolic pathways of Eimeria spp. (Looker et al., 1986; Kant et al., 2013). Anti-coccidial drugs have reduced the treatment cost per bird significantly, increased the stocking density of birds in a single house, improved the quality of the end products, consequently, remained as the most popular coccidiosis treatment in larger poultry industries (Allen and Fetterer, 2002; Price, 2012). There are three major types of anti-coccidial drugs based on their origin and specific mode of action as follows (Allen and Fetterer, 2002; Peek and Landman, 2011):
-
Synthetic compounds
-
Ionophores/polyether antibiotics
-
An amalgamation of either two synthetic compounds (i.e., Meticlorpindol) or synthetic compounds and ionophore (i.e., Nicarbazin)
Chemically synthesized drugs (chemicals) that are capable of preventing coccidiosis infection are known as synthetic compounds (Peek and Landman, 2011). Sulphaquinoxaline, a sulphonamide chemical compound was first used as the anti-coccidial agent to prevent coccidiosis of poultry in 1948 (De Gussem, 2007). Those synthetic compounds diminish the Eimeria parasites in the host by either inhibiting their folate synthesis pathways, mitochondrial respiration, thiamin uptake or also through the unknown mode of actions (Noack et al., 2019).
Sulfonamides (Fig. 14) inhibit the cellular replication of the Eimeria parasite (especially in Eimeria brunetti and Eimeria maxima) by limiting the presence of para-aminobenzoic acid (PABA) which is known to be involved in the production of folic acid during the schizonts and sexual stages of the life cycle (Lebkowska-Wieruszewska and Kowalski, 2010; Peek and Landman, 2011).
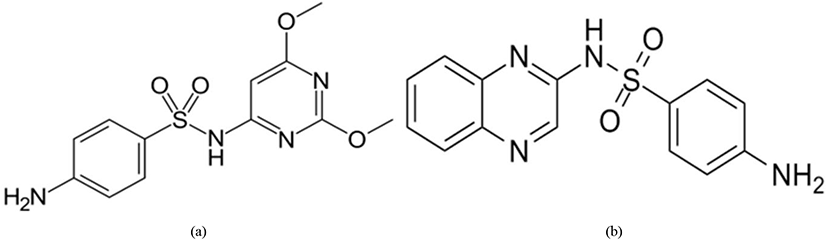
The anti-coccidial activity of ethapobate (Fig. 15) is identical with the sulphonamides, as it interferes with PABA to inhibit the folate production of the parasite cell which is highly productive for Eimeria maxima and Eimeria brunetti (Peek and Landman, 2011; Noack et al., 2019).
Clopidol (Fig. 16) is a complete coccidiostat that inhibits mitochondrial respiration and ATP formation in the early stages of Eimeria parasites (i.e., sporozoites and trophozoites) (Anon, 2010; Kant et al., 2013).
Quinolone is a group of an artificially synthesized drugs (comprised of decoquinate and methyl benzoquate; Fig. 17) that inhibits mitochondrial respiration and ATP formation of the Eimeria parasites by altering the electron transportation system (Noack et al., 2019).
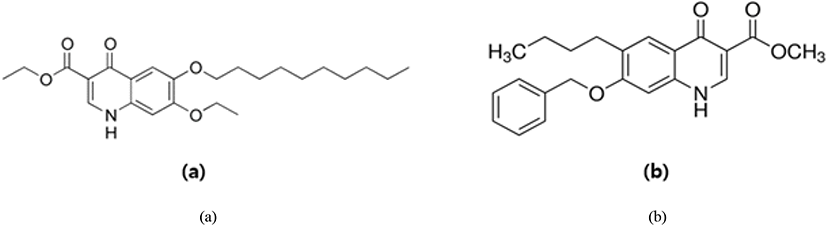
Amprolium hydrochloride (Fig. 18) is one of the safest synthetic anti-coccidial drugs used to act against the trophozoites and schizonts of Eimeria parasites (especially against E. acervulina, E. necatrix, E. tenella) and it leads to suppress the gametogenic stage and sporulation of the oocysts (Kant et al., 2013). Amprolium inhibits the production of thiamine pyrophosphate in Eimeria parasites by limiting the free thiamine (amprolium is a thiamine antagonist) that consequent in disturbed metabolic reactions (James, 1980; Noack et al., 2019).
Ionophores/polyether antibiotics are lipid-soluble compounds (with multiple cyclic ether groups) mainly produced as a by-product from the fermentation process of Streptomyces spp. or Actinomadura spp. that elucidates coccidiocidal properties during the sexual and asexual stages of Eimeria life cycle (Shivaramaiah et al., 2014; Quiroz-Castañeda and Dantán-González, 2015; Noack et al., 2019). Ionophores impair the vital transportation of metal cations and natural Na/K concentration gradient across the cell membranes that amplifies the Na+ and Ca2+ ion concentration in the parasite plasma. It leads to retarded development of sporozoites, as well as poor membrane integrity, increased osmotic pressure, elevated intracellular pH, cytotoxicity and inhibition of substrate oxidation with ATP hydrolyzation in parasitic mitochondria that resulting in the eventual death of the cell (Kant et al., 2013; Antoszczak et al., 2019).
Despite the destruction of Eimeria parasites, polyether antibiotics develop the immunity of the host against coccidiosis with a slow rate of drug resistance as well as improved growth performance, thus considered the most effective and successful method to control coccidiosis in recent history (Chapman, 1999; Kala et al., 2014). Generally, three types of polyether antibiotics can be identified based on their structure, affinity towards cations, and transport rate capacity as follows (Dowling, 1992; Peek and Landman, 2011):
-
Monovalent ionophores (affinity towards monovalent cations)
-
Divalent ionophore (affinity towards divalent cations)
-
Monovalent glycosidic ionophores (no specific affinity towards monovalent or divalent cations) (see Table 6)
Type of the ionophore | Name of the ionophore and molecular formula | Produced organism | Associated metal ions | References |
---|---|---|---|---|
Monovalent ionophores | Salinomycin (C42H70O11) | Streptomyces albus | K+, Na+, Cs+ | Antoszczak et al. (2019), Noack et al. (2019) |
Monensin (C36H62O11) | Streptomyces cinnamonensis | Na+, K+, Rb+, Cs+, Li+ | Liu and Hermann (1978), Rutkowski and Brzezinski (2013) | |
Narasin (C43H72O11) | Streptomyces aureofaciens | K+, Na+ | Jeffers et al. (1988) | |
Divalent ionophores | Lasalocid (C34H54O8) | Streptomyces lasaliensis | Ba+2, Sr+2, Ca+2, Mg+2 | Rutkowski and Brzezinski (2013) |
Ionomycin (C41H70CaO9) | Streptomyces conglobatus | Ca+2, Mg+2, Ba+2, Sr+2 | Liu and Hermann (1978), Antoszczak et al. (2019) | |
Monovalent glycosidic ionophores | Maduramicin (C47H80O17) | Actinomadura yumaensis | Na+, Mg+2 | Liu et al. (1983), Gutiérrez et al. (1999), Maron et al. (2016), Noack et al. (2019) |
Semduramicin (C45H76O16) | Actinomadura roserufa | Na+, Ca+2 | Dirlam et al. (1992), Rychen et al. (2018) |
Phytogenic compounds/phytochemicals include the extensive range of plant-derived bioactive composites that have been used as prophylactic agents in improving the performance of chickens, as well as, eliminating coccidiosis or alleviating its deleterious effects (Shivaramaiah et al., 2014; Yang et al., 2015; Irivboje et al., 2020). supplementation of phytochemicals to the poultry diet can be undertaken either as essential oils or herbal extracts, to provide antiparasitic, antimicrobial, antiviral, and antioxidative properties to the diet (Brenes and Roura, 2010; Puvača et al., 2013). At present, the usage of phytochemicals for preventing poultry coccidiosis have been promoted over the other traditional methods (i.e., vaccination, use of anti-coccidial drugs) due to its safe nature, no development of drug resistance and no drug residual accumulation in the host that certified a safe end product to the consumer (Abbas et al., 2012; Allouiet et al., 2014; Giannenas et al., 2014). Several authors have identified a great number of phytogenics to act against Eimeria spp. in poultry and frequently used compounds are mentioned in Table 7.
Bioactive compound/s name | Principle plant species/s | References |
---|---|---|
Carvacrol | Origanum vulgare | Alagawany et al. (2015) |
Thymus vulgare | Alagawany et al. (2015) | |
Lepidium flavum | Alagawany et al. (2015) | |
Thymol | Thymus vulgare | Yitbarek (2015) |
3,4,5-trihydroxybenzoic acid | Rheum officinale | Zhang et al. (2018) |
Eucalyptus robusta Smith | Zhang et al. (2018) | |
Semen corni | Zhang et al. (2018) | |
Gingerdoine, Gengerdiol and Gingerol | Zingiber officinale | Ali et al. (2019) |
Diallyl disulfide, Alliin and Alliciin | Allium sativum | Ali et al. (2019) |
Eugenol | Syzygium aromaticum | Evans et al. (2001) |
Menthol | Mentha x piperita L. | Evans et al. (2001) |
Anethol | Pimpinella anisum | Yitbarek (2015) |
Cinnamaldehyde | Cinnamomum verum | Orengo et al. (2012) |
Betaine | Beta vulgaris sp. | Kumar et al. (2014) |
Curcumin | Curcuma longa | Kumar et al. (2014) |